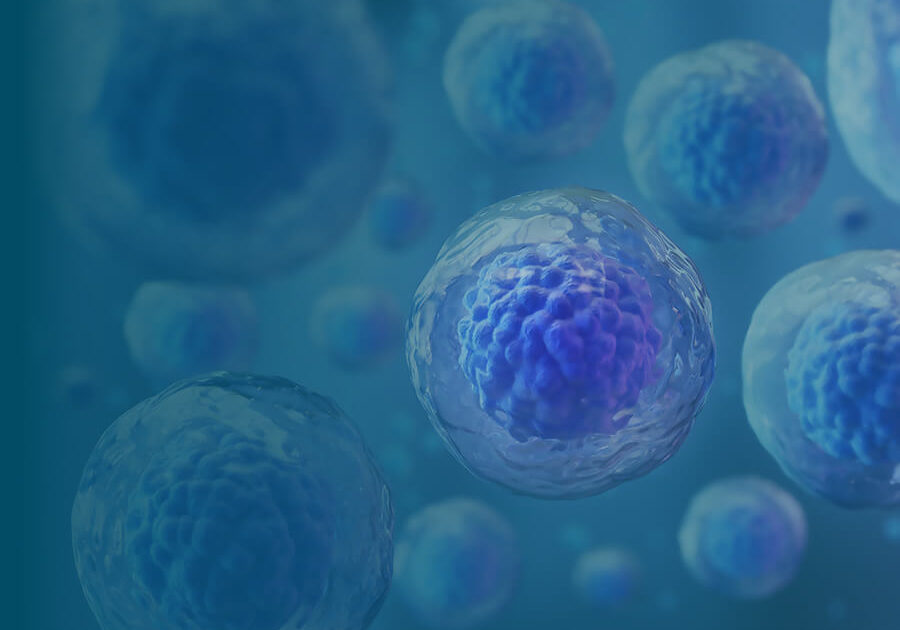
Pluripotent Stem Cells: How to Best Use Them in Your Research
Pluripotent stem cells, whether embryonic or induced (ES cells and IPS cells) are an attractive option for cell therapy as they can proliferate infinitely and differentiate into any tissue.
Scientists started to use mouse-derived embryonic stem cells in the early 1980s, and it was only in 1998 they were able to derive the first pluripotent cells from human embryos.
The next breakthrough in stem cell research came in 2006, when researchers identified the conditions required to genetically reprogram certain differentiated cells into a stem cell-like state.
What are Pluripotent Stem Cells and Where Can They be Found? (1)
Pluripotent stem cells are undifferentiated cells (“yet-to-develop” into their final destiny). able to proliferate and mature into the three germ layers that form a human being– endoderm, mesoderm, and ectoderm – giving origin to several tissues and organs. There are two kinds of pluripotent stem cells: embryonic stem cells (ES cells) and induced pluripotent stem cells (iPS cells).
ES cells are derived from embryos at a very early developmental phase when the “yet-to-develop” cells form the inner cell mass (ICM) of the blastocyst.
While at this stage the cells will lose their unlimited differentiation potential, if harvested and cultured under the right condition, they can replicate indefinitely and maintain their potential to develop any type of body tissue.
In contrast, iPS cells can be derived from almost any somatic tissue as they can be reprogrammed. Reprogramming entails the expression in a somatic cell of a set of core pluripotency-related transcription factors: OCT4, SOX2, KLF4, and MYC that can reprogram the cell back into an embryonic-like pluripotent state (2) and differentiate into the desired cell type.
Why are Pluripotent Stem Cells Important?
Embryonic and induced pluripotent stem cells ( IPS cells) have the potential to provide unprecedented opportunities for therapies against intractable diseases and devastating injuries.
They have been explored as a treatment for severe and painful human ailments such as spinal cord injuries, Type I Diabetes, age-related macular degeneration, Parkinson’s disease, amyotrophic lateral sclerosis, Alzheimer’s disease, heart disease, stroke, burns, cancer, and osteoarthritis.
With their potential for unlimited proliferation, ES cells and IPS cells are a vast source of potentially any type of human cell, but they come with several challenges. Let’s walk through some of them.
The Challenge – and Art – of Maintaining Pluripotency in Culture
Culturing pluripotent stem cells is a delicate art.
The environment needs to be tightly controlled and the cells need to be checked at each stage to ensure that they are differentiating as desired.
Reprogramming methods can vary throughout the field, including how cells are passaged and what media is used. Somatic reprogramming methods also have varying levels of success. Cell viability is another top concern for scientists working with stem cells.
Stem cells are fragile and gene editing or freeze-thaw cycles can impact cell survival. Culture conditions also need to be sterile; mycoplasma contamination is commonplace in many labs.
Mycoplasma compete with stem cells for nutrients and can have a profound impact on global gene expression levels within the cells. Finally, varying culture methods and practices can cause line-to-line variability; there is a need for isogenic experimental controls.
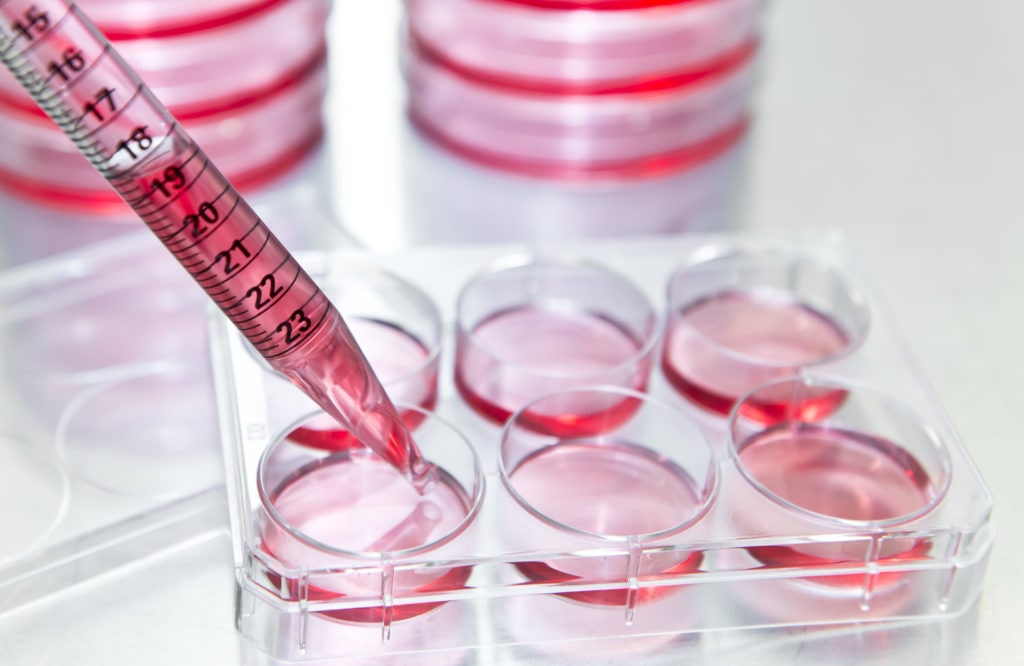
Tumorigenesis of Pluripotent Stem Cells
If even a small number of “yet-to-develop” and/or immature cells are retained in the final differentiated cell product, cancer and teratomas may emerge: ESC cell lines are heterogeneous and have different efficiencies of differentiation.
In mouse models, IPS cells cell lines showed defects in differentiation, with some differentiating at 95% efficiency while others differentiated at 80%, leaving many residual undifferentiated cells (3). When these “yet-to-develop” cells are transplanted in immune-deficient mice, they result in teratoma formation.
There are ways to obviate this occurrence:
- Improving the efficiency of in vitro reprogramming methods aimed at reducing the number of remaining undifferentiated cells (4).
- Using a more stringent method of purification, like a GMP-compatible cell sorting system, to enable the purification of mature differentiated cells: it can be by negative selection using anti-CD30 antibody because CD30 is expressed on undifferentiated iPSC only, or by double purification, with negative selection followed by positive purification (5)
Another common risk when working with iPSCs – as with any other in vitro expanded cell – is that expansion inevitably causes genetic alterations, such as chromosomal abnormalities, copy number variations, and single nucleotide mutations.
While chromosomal abnormalities can in some cases be avoided by subcloning error-free cells, copy number variation and SNPs can be monitored by qPCR, SNP Arrays, or even Whole Genome Sequencing (WGS) (4). However genetic alterations are still hard to interpret since there is still no complete consensus on which genetic mutations will definitively cause cancer (6), which further complicates the risk analysis needed before starting clinical trials.
Immunogenicity of Pluripotent Stem Cells
iPSCs can be autologous i.e., harvested from the patient or allogeneic – derived from donors.
Even if autologous transplanted pluripotent stem cells don’t cause rejection (4), the allogeneic approach is still preferred. Producing medical-grade pluripotent stem cells is time-consuming, and in the case of an acute injury like heart failure or severe spinal injury, the time needed to produce an autologous product would be detrimental to the patient. Allogeneic products can be made available “off-the-shelf” and are thus the optimal choice despite the risk of rejection.
The immunological reactions, including rejection, triggered by the histoincompatibility of the allogeneic product are overcome with the use of immune suppressant drugs. In immune-privileged tissues like the central nervous system or eyes, immune suppressants can be successfully tapered off with a low chance of rejection.
HLA Biobanks and HLA Cloaking
How can scientists overcome immunological barriers and standardize the use of pluripotent stem cells?
A well-known method is matching the HLA haplotype, an approach widely used in hematopoietic stem cell transplantation, where a full or almost full HLA match between donor and recipient is required. To extend the search for histocompatible donors, worldwide bone marrow banks have been created for millions of registered donors. A collaboration with these biobanks would facilitate the identification of suitable donors whose blood samples may be collected for iPSC generation.
Another more recent approach is HLA cloaking, which involves gene-editing technologies like CRISPR.
The HLA genes in the pluripotent stem cells can be inactivated: HLA-A, B, and C by deleting their common component, beta-2-microglobulin or B2M; HLA-DP, DR, and DQ by deleting one of the 4 trans-activators essential for their transcription.
However, MHC-I molecules are ubiquitously present on cell surfaces and contribute to self-tolerance by binding to the inhibitory receptors on NK cells. By cloaking the HLA-I genes, self-tolerance is broken, and stem cells are targeted and lysed by NK cells. There are several strategies to overcome this, from introducing chimeric molecules that bind to NK cells without causing immunogenicity, to selectively cloaking only HLA genes critical to immune rejection (7,8).
With these strategies, it should be possible to create universal cell lines for all recipients, although there is much research that needs to be done to optimize and understand these approaches.
The nCounter® Stem Cell Characterization Panel
Recognizing the challenges associated with culturing stem cells and the need for standardization across the culturing process, NanoString has developed a comprehensive gene expression panel focused on stem cell characterization. The nCounter Stem Cell Characterization Panel enables scientists to deeply characterize ES cells and iPS cells, optimizing research on these cells.
You can evaluate viability, confirm functionality, and determine pluripotency with a single robust, automated, and reproducible assay. You can also assess stem cell health during production and easily detect mycoplasma contamination to quickly optimize cell culture conditions and expedite time to results. The introduction of this panel gives the stem cell field an opportunity to better standardize and streamline research, ultimately accelerating discoveries.
Do you want to learn more about this fascinating subject? These top scholarly articles and research papers can help you learn more about pluripotent stem cells.
For Research Use Only. Not for use in diagnostic procedures
Bibliography
- https://pubmed.ncbi.nlm.nih.gov/33007237/
- https://www.nature.com/articles/cr200759
- https://pubmed.ncbi.nlm.nih.gov/24259714/
- https://www.nejm.org/doi/full/10.1056/NEJMoa1608368
- https://www.nature.com/articles/s41598-018-21923-8
- https://pubmed.ncbi.nlm.nih.gov/30293088/
- https://pubmed.ncbi.nlm.nih.gov/28504668/
- https://www.cell.com/cell-stem-cell/fulltext/S1934-5909(19)30047-5