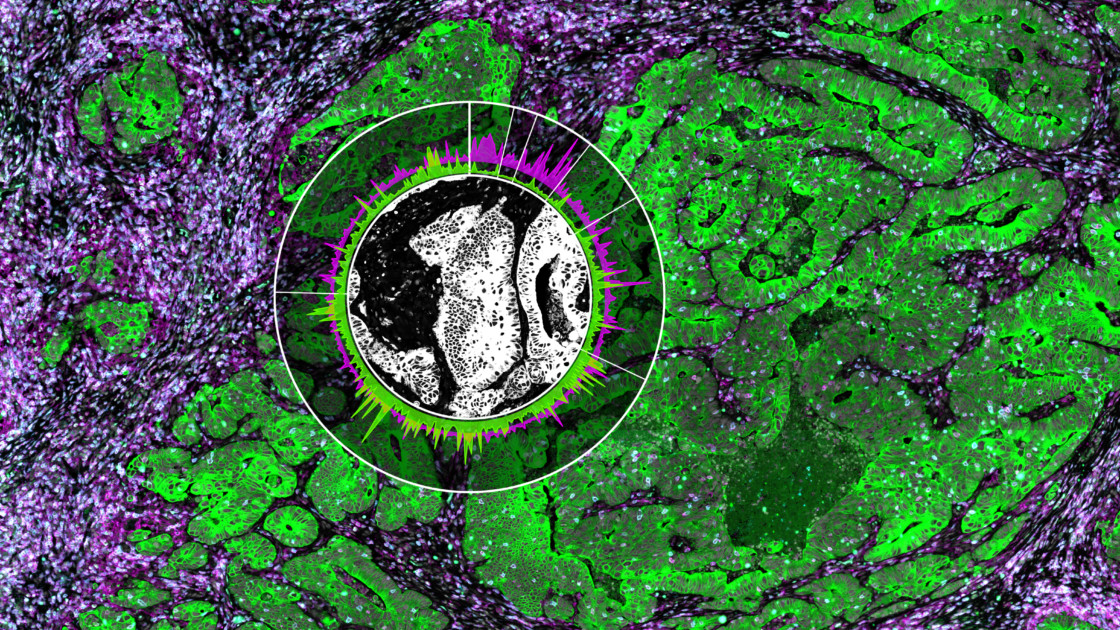
Spatial Transcriptomics Overview
What is spatial gene expression?
Spatial gene expression examines transcriptional dynamics through the lens of location within tissue and/or transcriptional dynamics between unique cells within a tissue. With spatial gene expression, users get additional insights into cellular biology as they can get gene expression information within a given three-dimensional cellular context.
Gene expression refers to the molecular process of taking information from the genome (DNA), creating an mRNA copy, then translating that mRNA copy into a protein. These proteins, or in some cases RNA species, go on to cause a biological change in the organism. Gene expression can vary widely between cell and tissue types. The specific sequence and type of gene expression are essential for distinguishing different cell types and differentiation along distinct cellular lineages.
Spatial gene expression refers to examining transcriptional genomics in its spatial context. Spatial context can refer to two-dimensional or three-dimensional space within a tissue, cell, or at subcellular resolution.
Cells exist in an organism in three-dimensional space. A cell’s social and spatial context is often critical for proper functioning. Therefore, assessment of gene expression in context is important for a more thorough view of the molecular biology of cells.
Scientists can determine spatially resolved gene expression using a combination of two standard molecular biology techniques, immunofluorescence and gene sequencing. For example, researchers can generate spatial gene expression using fluorescently labeled antibodies or probes to detect proteins or RNAs. Once a region of interest is identified, the user can shine UV light on the region, causing a tag to be cleaved from the antibodies or probes. These tags can then be collected then sequenced using next-generation sequencing.
Why is spatial gene expression important?
Spatial gene expression is the visualization of gene expression patterns in three dimensions within cells in their native state. Biology is inherently spatial, and individual cells do not function in isolation but work together forming a complex network of gene interactions spatially to organize themselves and communicate with their surroundings.
The spatial profile of each gene within a cell is tightly linked to the biological functions it drives.
Localization of gene expression with a positional context and understanding how transcriptional dynamics vary within such context is crucial to interpreting various biological, physiological, and pathological processes occurring in a single cell or tissue.
For example, in a developing embryo, the correct deployment of regulatory genes for cell differentiation that leads to tissue patterning and organ formation is dependent on the location, timing, and level of gene expression. Dissecting these complex gene regulation networks spatially is necessary to understand the process leading to organogenesis. Another advantage of spatial gene expression is that it can be quantified, further enhancing our understanding of gene regulation.
Analysis of highly heterogeneous cancer tumors is another area that benefits tremendously from the knowledge of spatial gene expression enabling the study of the tumor and immune cell interactions, cell heterogeneity in tumors, or identifying specific biomarkers beneficial for clinical prognosis.
Traditionally, spatial gene expression patterns were captured using in situ hybridization or immunohistochemistry techniques. While these techniques did provide spatially resolved data, quantification was often difficult, and the analysis was limited to only a handful of genes in a single experiment.
Today, several advanced high-resolution spatial profiling technologies are available based on in situ hybridization and in situ sequencing methods. These technologies are fully automated and can accurately localize and quantify spatial gene expression with high throughput, looking at tens of thousands of genes localized in a small section of tissue within a single experiment. Many of these technologies use specialized molecularly barcoded probes that bind mRNA on tissue sections that are then counted, sequenced, and amplified to capture and measure all the gene activity in cells and tissues.
What does spatial transcriptomics do?
Spatial transcriptomics is a method that enables researchers to spatially localize and quantify gene expression in the form of mRNA transcripts within cells or tissues that are in their native state. Spatial transcriptomics offers an unbiased exploration of mRNA transcripts in situ that can be quantified.
All cells in a multicellular organism harbor the same genome; however, the genome’s expression pattern determines the physiological fate of the cell. This varying gene expression pattern drives cellular diversity, and with the use of spatial transcriptomics, mapping gene expressions with positional context is now possible. Before the advent of spatial transcriptomics, cells and tissues were dissociated and homogenized to isolate RNA transcripts, thus losing spatial context in the process. Thus, spatial transcriptomics can be utilized to generate transcriptome maps and atlases based on distinctive gene expression profiles and connect them to their cellular location and morphology, quantify differences in gene expression among cells, and provide insights into the cause and effect of diseases.
Spatial transcriptomics technologies – such as the GeoMx® Digital Spatial Profiler, are fully automated and use specialized probes that bind mRNA on tissue sections to capture the whole transcriptome, displaying numerous genes localized spatially within a single experiment. The RNA bound by probes is molecularly barcoded and can then be utilized to synthesize complementary DNA (cDNA) for Next Generation Sequencing (NGS) libraries generation or quantified using the standard NanoString nCounter®. Finally, the data is visualized using a range of computational tools to quantify and localize gene expression.
What is single-cell spatial transcriptomics?
Single-cell spatial transcriptomics is the analysis of mRNA expression profiles with spatial context at the level of a single cell. Each cell has a unique transcriptomic fingerprint as gene expression patterns can be heterogeneous even amongst similar cells in both standard and abnormal cell states.
Thus, the application of single-cell spatial transcriptomics is valuable for understanding more nuanced spatial variables of gene expression such as enrichment of ligand-receptor expression at the interface of interacting cells or cell lineage relationships in development.
The most used technology to date to measure mRNA transcripts within each cell is the single-cell RNA sequencing (scRNA-seq) technique. But a major drawback of these technologies is that they fail to capture positional information of the RNA transcript as they require tissue dissociation and cell isolation. On the other hand, spatial transcriptomics techniques use intact tissue sections, spatial barcoding, or in situ hybridization to retain positional information and can be categorized into two groups: profilers that resolve high throughput data, often at the level of the whole transcriptome but do not have single-cell resolution, and imagers that provide single-cell and subcellular resolution using in situ reagents but with a lower throughput than the profilers.
Single-cell imagers use sequential cycles of probe hybridization and imaging and offer the potential to combine the benefits of scRNA-seq analysis with added spatial resolution at single-cell or even subcellular resolution. A recently developed technology for spatial single-cell imaging, the CosMx Spatial Molecular Imager (SMI), is an integrated system with mature cyclic fluorescent in situ hybridization (FISH) chemistry, high-resolution imaging readout, interactive data analysis, and visualization software. This enzyme-free, amplification-free, hybridization-based single-cell transcriptomics technology can detect about a thousand different types of RNA transcripts spatially at a resolution of <50 nm.
For Research Use Only. Not for use in diagnostic procedures.
The CosMx™ SMI and decoder probes are not offered and/or delivered to the Federal Republic of Germany for use in the Federal Republic of Germany for the detection of cellular RNA, messenger RNA, microRNA, ribosomal RNA and any combinations thereof in a method used in fluorescence in situ hybridization for detecting a plurality of analytes in a sample without the consent of the President and Fellows of Harvard College (Harvard Corporation) as owner of the German part of EP 2 794 928 B1. The use for the detection of cellular RNA, messenger RNA, microRNA, ribosomal RNA and any combinations thereof is prohibited without the consent of the President and Fellows of Harvard College (Harvard Corporation).