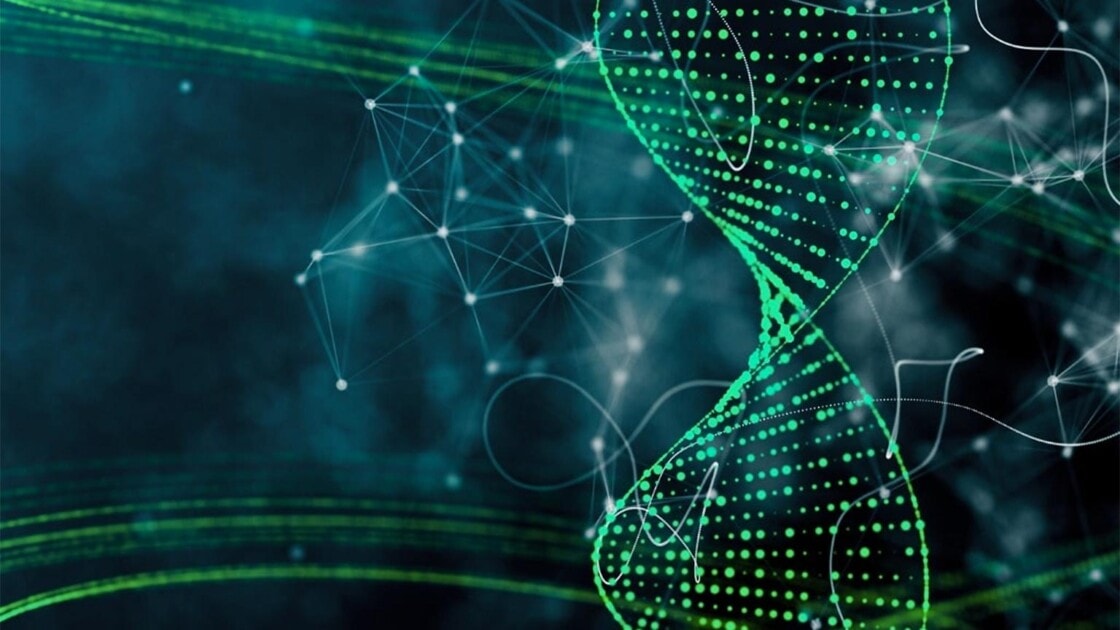
Why Oligonucleotides Are Critical Components of DNA and RNA Expression Research
With all the advances in molecular biology over the last several decades, one of the most consistently used tools is the oligonucleotide. Oligonucleotides are relatively short (oligo) single-stranded sequences of nucleic acid subunits (nucleotides), the monomeric components of both DNA and RNA. The synthesis and use of oligonucleotides (sometimes referred to as oligomers or just “oligos”) has been invaluable for many key molecular biology techniques, including PCR, DNA sequencing, labeled probes, plasmid construction, and genomic manipulations. The state of biological research and biomedicine would not be where it is today without the use of oligonucleotides.
Although oligonucleotides are relatively short strands of nucleotides, they can still be up to 100 nucleotides, or base pairs (bp), long. Typical oligos used in research range from 5-50 bp. While 100 bp may not seem short, it is when compared to the sizes of genomes (ranging from 2 kilobase [kb] in viruses to 100,000 megabase [Mb] in some plants) and the other nucleic acid research workhorse, the DNA plasmid (ranging from 2 kb to 500 kb). Therefore, even a 100 bp oligo constitutes a very small percentage of the size of most DNAs under study. Most RNAs are also considerably bigger than oligos, with mRNAs ranging from 300 to 10,000 bp and rRNAs are between 1.5 kb and 4 kb. However, some naturally occurring RNAs are similar in size to synthesized oligonucleotides – tRNAs are between 70 and 100 bp and snRNAs are less than 200 bp. Even at these smaller sizes, it is possible to design oligos to study these RNAs.
Why oligonucleotides are valuable for biology research
The utility of oligonucleotides for nucleic acids research stems from a few of their properties – relatively chemically stable, easily manipulated, and easily labeled for visualization. Complementarity and sequence specificity are key properties of oligos. Complementarity is the chemical recognition between nucleotide bases that drives specific pairs to align with each other through hydrogen-bonds, enabling the formation of double-stranded molecules. For DNA, complementary bases are adenine (A) and thymine (T), and guanine (G) and cytosine (C). For RNA, the set up is similar, though uracil (U) is substituted for thymine. The process of complementary bases finding each other to form double-stranded molecules through hydrogen-bonding is called annealing. All nucleic acids have this capability, which is why DNA can be used a probe for RNA.
Oligonucleotides are valuable as probes in part because their complementarity promotes sequence integrity and specificity.
Complementarity is key to information transfer between nucleic acid molecules, and the specific hydrogen bonding between complementing nucleotides dictates sequence specificity, since each nucleotide in a single-stranded sequence dictates that its complementary nucleotide be added to newly produced strands. Complementarity also preserves sequence integrity for this same reason. Therefore, promotion of sequence integrity and specificity by complementarity is what makes oligonucleotides valuable as probes and other molecular tools.
The early days of oligonucleotide work
Attempts to synthesize DNA strands in the laboratory were done as early as 1955, soon after the establishment of the structure of DNA; however it took until 1968 for a laboratory to synthesize a full gene sequence (Khorana). Perfection of the process continued over the next decade. An early use of synthesized oligonucleotides was in a study of whether complementary (also known as antisense) sequences could be used to disrupt specific protein production in the Rous sarcoma virus (Zamecnik). The ability to label oligonucleotides for visualization, first using radioactive isotopes incorporated in the oligo itself, and subsequently by chemically detection and fluorescent methods, led to broader use of oligos as probes and primers for a variety of techniques.
Oligonucleotide primer use in PCR
PCR (polymerase chain reaction) is a method used to rapidly amplify the number of copies of a specific target DNA sequence from a sample. As mentioned, the DNA polymerase used to amplify the target needs primers to work. When DNA is the target molecule, both forward and reverse primers are needed to make double-stranded DNA product. Oligonucleotide primers are designed to anneal to highly specific complementary sequences in the target. Factors taken into consideration when designing PCR primers include the melting and annealing temperature of the selected sequence, and the uniqueness of the regions where the primers bind to ensure the correct gene is amplified. In this way scientists can amplify DNA from a very small sample to obtain enough material to study.
Use in DNA sequencing
Oligonucleotide primers are also used for DNA Sequencing. Primers for sequencing have similar requirements as those for PCR, such as melting and annealing temperatures, sequence specificity, and region of hybridization, though a different set of parameters is used due to different polymerase reaction conditions.
Use with plasmids
Oligonucleotides are also used in construction of plasmids, small circular naturally occurring DNA molecules in bacteria and other microorganisms that are widely used as vectors for transferring engineered genetic information into cells. Other techniques employing engineered plasmids containing oligonucleotides include the replication and expression of recombinant DNA sequences and characterization of gene regulatory sequences. Plasmids are also used in genome manipulations, often involving insertion of an oligonucleotide into a gene of interest to disrupt its expression so to study its function.
Oligonucleotide probes
Another common use of oligonucleotides is as probes for detecting specific RNA or DNA sequences of interest. Oligonucleotide probes are designed to be complementary to the sequence of interest and labeled with radioactive or fluorescent molecules, though other methods are available (such as digoxigenin).
These probes are denatured to form single stranded DNA (ssDNA) that can hybridize to the target, which is typically immobilized on a membrane or in situ. Detection of the hybridized labeled probe can be used either for localization or quantitation of the target sequence. Probe specificity can be modified by adjusting the hybridization conditions (such as temperature and buffer conditions) for higher or lower stringency. High stringency conditions permit hybridization only between highly similar sequences, whereas less similar sequences can hybridize under low stringency.
Oligonucleotide probes, both DNA- and RNA-based, are routinely used to screen gene libraries, detect nucleotide sequences with blotting methods, in situ hybridization, gene expression analysis, and tissue microarrays, methods that continue to move research forward.
Novel Uses of Oligonucleotides
Novel applications have recently been developed using oligonucleotides. Two of the more prominent ones are aptamers and antisense oligonucleotides.
Use in aptamers
Aptamers are artificial oligonucleotides designed to bind one or more specific targets and can be used in many of the same applications as antibodies. Optimized to achieve specific and sensitive binding to a chosen target, among a variety of other beneficial features, aptamers have been used as drugs and drug delivery systems and in identifying molecular markers of disease. Aptamers can be designed to change their shape dramatically when binding their target, making them useful as molecular switches for various purposes, including as part of some biosensors (Li).
Use in ASOs
Another recently developed application employs antisense oligonucleotides (ASOs) to target mRNAs involved in disease processes. Known as antisense therapy, this form of treatment uses ASOs to alter mRNA expression through various mechanisms, including ribonuclease-mediated decay of the pre-mRNA, direct steric blockage, and modulation of exon sequence by binding to the splice site on pre-mRNA. Antisense therapy has proved effective against several diseases, leading to approval of ASOs for treatment of Duchenne muscular dystrophy and spinal muscular atrophy, among others, in the United States, the European Union, and elsewhere (Kim).
Use in molecular barcodes
The ability to design oligonucleotides with any custom sequence is an appropriate model for connecting the identify of target molecules to that sequence. Known as DNA barcodes (or sometimes molecular barcodes), the sequence information and specific hybridization capabilities of DNA mean that DNA oligonucleotides make useful barcodes.
The idea to use oligos of specific sequence for identification purposes was inspired by unique naturally occurring sequences that were identified as a means of definitively assigning organisms in the wild to a species. Engineered DNA barcodes incorporate unique sequences into experimentally relevant DNA for cellular and molecular identification purposes.
The unique design of oligonucleotide DNA barcodes provides a reliable means of identifying sequences of interest from a pool of DNAs.
Uses of lab-derived DNA barcodes include: inserting barcode sequences into libraries of mutants for reliably identifying mutants from pools of DNAs; inclusion of barcodes in cDNA libraries to allow pooling of DNA from independent samples for high-throughput sequencing; and inclusion in construction of an insertional mutations viral vector library as a means of detecting and identifying the insertions in phenotypically mutant yeast (Chen). The unique design of oligonucleotide DNA barcodes provides a reliable means of identifying sequences of interest from a pool of DNAs.
Oligonucleotides for high-throughput gene expression analysis
Oligonucleotide molecular barcodes are also used during gene expression profiling for tagging mRNA transcripts in tissues (DNA barcoding). NanoString pioneered a unique chemistry for use in its nCounter® Pro Analysis System that uses both DNA and RNA oligos to form multi-part probes that identify and quantitate transcripts present in the tissue of interest.
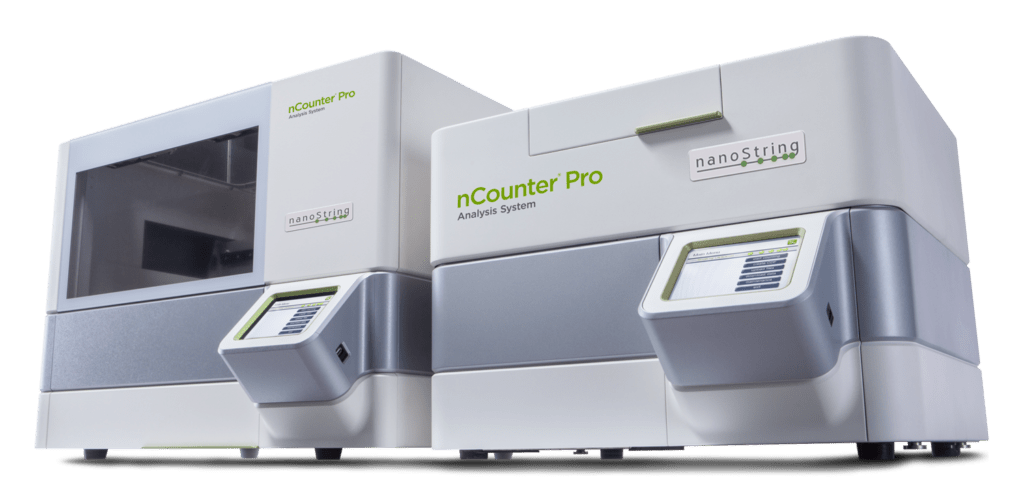
Three specifically-designed unique oligonucleotides constitute the heart of each nCounter probe. Based on the target gene sequence, two 50mer DNA oligos are designed to complement opposite ends of a unique 100 bp stretch of the gene. Attached immediately adjacent to one 50mer oligo is an additional DNA oligo sequence, known as the backbone, that anneals to a complementary RNA molecular barcode. Individual segments of the RNA oligo are labeled with specific fluorophores arranged in various specific linear orders to create a unique code for each gene of interest (Geiss). This DNA-RNA hybrid molecule constitutes the reporter half of the probe pair. The second half of the two-part probe consists of the second gene-specific sequence taken from the adjacent end of the 100 bp target sequence, forming the capture probe (Geiss).
Hybridization of the reporter and capture probes to the target RNA creates a tripartite structure identified by the specific fluorescent signal of the reporter probe, which can also be used for quantifying each transcript. Minimizing target sequence length to 100 bp gives these probes the specificity for reliable hybridization while providing a high tolerance for challenging sample types, suboptimal RNA quality and unequal copy numbers. The elegance of fluorescent molecular barcoded probes used in the nCounter technology, as well as the other examples presented here, demonstrate the priceless role oligonucleotide synthesis plays in modern biotechnology.
References
Chen BR, Hale DC, Ciolek PJ, Runge KW. Generation and analysis of a barcode-tagged insertion mutant library in the fission yeast Schizosaccharomyces pombe. BMC Genomics. 2012;13:161. Published 2012 May 3. doi:10.1186/1471-2164-13-161
Geiss GK, Bumgarner RE, Birditt B, Dahl T, Dowidar N, Dunaway DL, Fell HP, Ferree S, George RD, Grogan T, James JJ, Maysuria M, Mitton JD, Oliveri P, Osborn JL, Peng T, Ratcliffe AL, Webster PJ, Davidson EH, Hood L, Dimitrov K. Direct multiplexed measurement of gene expression with color-coded probe pairs. Nat Biotechnol. 2008 Mar;26(3):317-25. doi: 10.1038/nbt1385. Epub 2008 Feb 17. PMID: 18278033.
Khorana HG, Büchi H, Caruthers MH, Chang SH, Gupta NK, Kumar A, Ohtsuka E, Sgaramella V, Weber H. Progress in the total synthesis of the gene for ala-tRNA. Cold Spring Harb Symp Quant Biol. 1968;33:35-44. doi: 10.1101/sqb.1968.033.01.009. PMID: 5254575.
Kim YJ, Krainer AR. Antisense Oligonucleotide Therapeutics for Cystic Fibrosis: Recent Developments and Perspectives. Mol Cells. 2023 Jan 31;46(1):10-20. doi: 10.14348/molcells.2023.2172. Epub 2023 Jan 15. PMID: 36697233; PMCID: PMC9880599.
Li Y, Zhao J, Xue Z, Tsang C, Qiao X, Dong L, Li H, Yang Y, Yu B, Gao Y. Aptamer nucleotide analog drug conjugates in the targeting therapy of cancers. Front Cell Dev Biol. 2022 Dec 5;10:1053984. doi: 10.3389/fcell.2022.1053984. PMID: 36544906; PMCID: PMC9760908.
Zamecnik PC, Stephenson ML. Inhibition of Rous sarcoma virus replication and cell transformation by a specific oligodeoxynucleotide. Proc Natl Acad Sci U S A. 1978 Jan;75(1):280-4. doi: 10.1073/pnas.75.1.280. PMID: 75545; PMCID: PMC411230.